Risks of bridge collapses are real and set to rise — here’s why
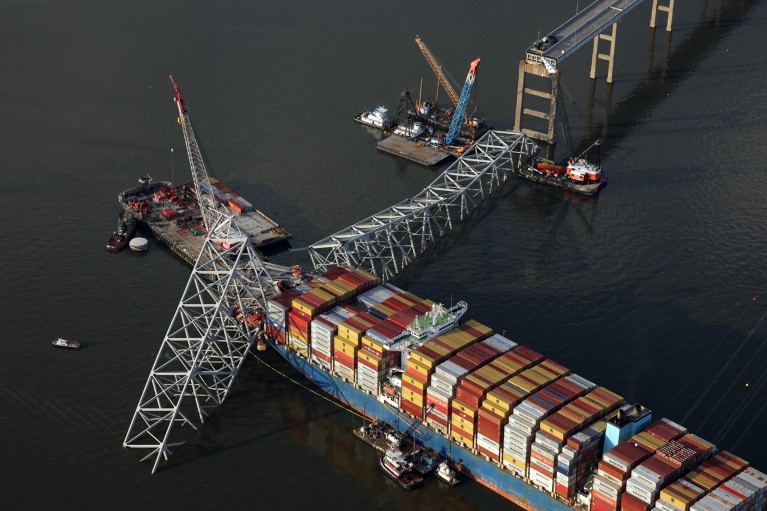
Six people in Baltimore, Maryland, were killed after a container ship crashed into and collapsed the Francis Scott Key Bridge in March. Credit: Kevin Dietsch/Getty
The catastrophic collapse of the Francis Scott Key Bridge in Baltimore, Maryland, on 26 March stunned the public and engineers alike. Six workers lost their lives after a container ship lost power and crashed into one of the bridge supports. The Port of Baltimore and the city’s road-transport network remain hobbled. And serious questions linger about the ineffectiveness of measures for preventing such disasters.
Although an identical scenario is unlikely to happen elsewhere, bridge collapses occur surprisingly often (see ‘Deadly bridge collapses’). In 2018, for example, part of the Polcevera Viaduct in Genoa, Italy, crashed to the ground during a rainstorm, killing 43 people. And many cases don’t make the headlines. In China between 2000 and 2014, for example, more than 300 highway bridge failures caused 564 fatalities and 917 injuries1.
Bridges are the most vulnerable and expensive assets of transportation networks. Beyond lives lost and the costs of replacement, the failure of a single bridge can hinder movement of people and goods in a region for months or years. Displaced traffic generates more congestion, pollution, road wear and accidents elsewhere. Delays in the delivery of goods disrupt global supply chains. Economic losses of bridge failures can mount to millions of dollars per day and spiral quickly2,3. Indirect losses (such as from increased travel time) can be 5–20 times greater than the direct losses4.
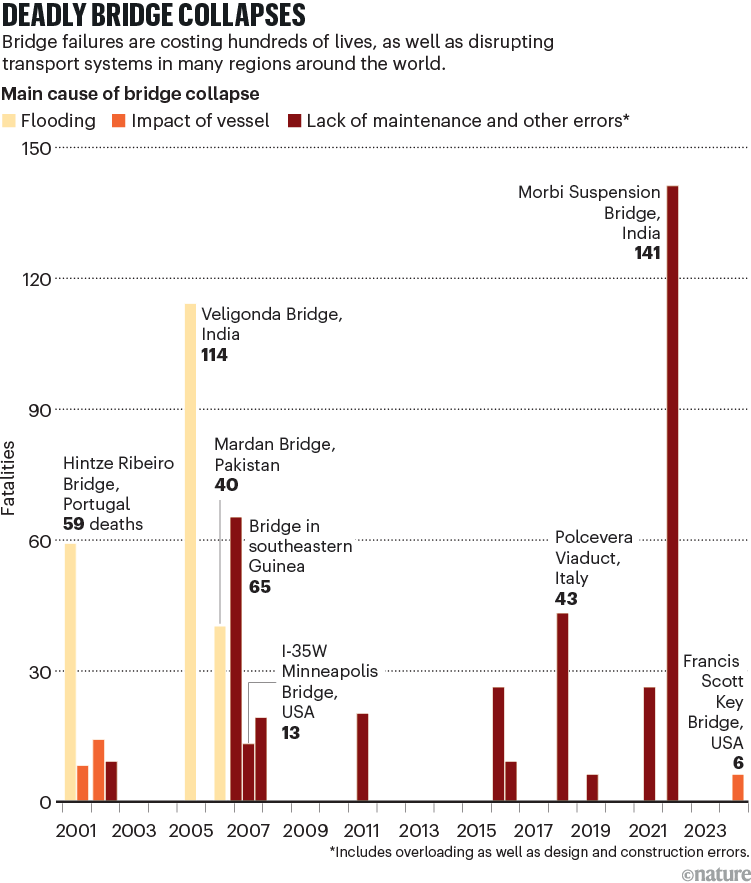
Source: Analysis by J. M. Adam et al.
Clearly, preventing bridges from collapsing is crucial. However, as bridges age, it becomes increasingly challenging to evaluate and ensure their structural safety. During the long service life of a bridge — typically 100 years — the vehicles and trains crossing them inevitably evolve, imposing loads that can exceed those considered when the bridges were conceived. Long-term changes to vehicles and traffic also expose bridges to collision risks that might not have been considered during design.
Deterioration of materials reduces the strength of structural components and is exacerbated by climate change and heat5–7. Extreme weather events that are more frequent and intense expose bridges to increased scour from water flow and more landslides and subsidence, with overall impacts that are difficult to predict8. Thus, ageing bridges are faced with greater risks and uncertainties that make it harder to evaluate their safety.
What needs to be done? Here we outline the problems and highlight how the world’s bridges can be made safer through better standards, maintenance, preparedness and lessons drawn from other industries.
Update standards
Current safety codes and standards still lack guidance on how to deal with the increasing risks of material deterioration and more frequent extreme events. Most codes focus on the design of new bridges, not on evaluating the safety of old ones. Yet the two scenarios raise different problems.
For example, designing the 1915 Çanakkale Bridge in Turkey, which opened in 2022, required optimizing aerodynamic performance. Ensuring the safety of the Clifton Suspension Bridge in Bristol, UK, which opened in 1864, requires protecting wrought-iron chain links that were manufactured for a previous bridge 180 years ago and understanding past safety interventions from the sparse information that is available.
A few national and international standards do address assessment and retrofitting of existing bridges — such as through technical specifications intended to be used with the first generation of Eurocodes, which are due to be updated next year (see go.nature.com/3wdrmes). However, these standards cover only general principles to assess current needs for structural interventions, not how to avoid bridge collapses in an uncertain future9.
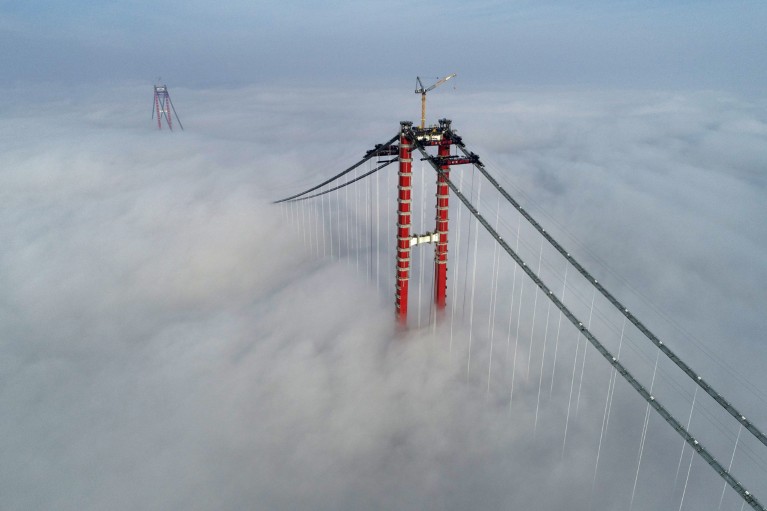
The design of the 1915 Çanakkale Bridge in Turkey, which opened in 2022, required aerodynamic modelling.Credit: Burak Akay/Anadolu Agency/Getty
Methods of evaluation also rely on historical data and trends to characterize loads due to traffic, vehicle impacts and environmental conditions. Yet, these vary widely through time and might not reflect future conditions.
The growing number of cars and trucks on roads means that bridges have to withstand a higher frequency of passage of vehicles, which can accelerate the growth of cracks and failures. Rail traffic is also increasing, as are the weight and length of freight trains. The load-carrying capacity of container ships docking in ports today has increased by almost 1,500% since 1968 (see go.nature.com/4dacdfp). The force imparted by such enormous vessels if they impact a bridge pier is unstoppable, as Baltimore tragically witnessed.
Global climate models also predict a wide range of possible future scenarios, casting more doubt on how today’s bridges will withstand extreme weather events over their lifetimes8.
Thus, bridge safety needs to be evaluated in a more holistic way, by combining comprehensive assessments of risks and data collected on existing bridges10.
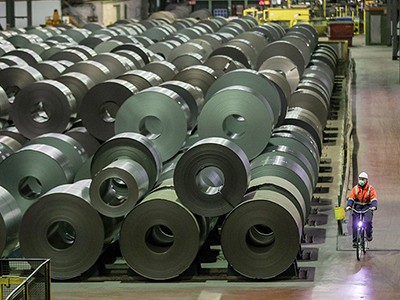
Cement and steel — nine steps to net zero
This will require four advances. One, more accurate methods to estimate environmental and operational loads that can cope with evolving predictions. Two, better models of how climate change will influence deterioration. Three, harmonized protocols for processing and interpreting data on the structural response of bridges. And four, dynamic decision-support tools that provide timely and relevant information to bridge managers.
Early efforts in this direction are under way. For example, through the International Association for Bridge and Structural Engineering (IABSE), bridge specialists are collaborating to propose safety assessment procedures that consider new hazards and to revise methods for assessing the reliability of existing structures. However, such developments are still not enough to change common practice.
Regulatory and standardization processes require agreements between many actors, from policymakers to practitioners. The European Commission is paving the way, and has committed to modernizing standards for the maintenance and control of European transport infrastructure, including bridges. To achieve this, it funded the IM-SAFE project (2020–23; go.nature.com/3qscerw) to review existing surveying and monitoring technologies and propose data flows for managing structures. Firm commitments from the commission, as well as acceptance of new codes by infrastructure managers, will be crucial for the successful design and release of a new standard in the next decade.
Repair worn bridges
The huge backlog of bridge repairs must be addressed urgently. In the United States alone, more than 46,000 bridges are considered structurally deficient, and some 178 million trips are taken on these bridges every day (see go.nature.com/3k41ztg). Repair costs have been put at US$125 billion. In Europe, at least 9,000 bridges require strengthening or repair11. These are alarming numbers, particularly considering how little data are available on the condition of bridges in other parts of the world.
As well as being cheaper than rebuilding, extending the lifespan of bridges brings environmental benefits. These include reductions in resource consumption, waste generation and carbon dioxide emissions.
Curb hazards and limit failure
Resilience, through better preparedness, is key to preventing catastrophic bridge collapses. In practice, that means that a transport system must be able to keep providing the service if a disruptive event occurs.
Engineers and bridge managers must develop a better understanding of the three stages that lead to structural collapse. These are: occurrence of a hazard; initial failure of a component owing to damage; and the propagation of failures through the structure. Various risk reduction measures are effective at each stage and can be used together to prevent collapse.
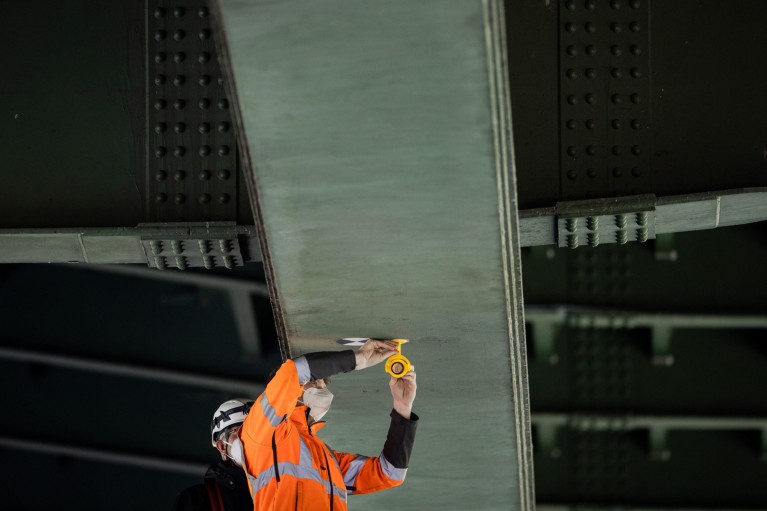
Engineers install sensors on a road bridge in Herne, Germany, after finding its girders had bent.Credit: Rolf Vennenbernd/dpa/Alamy
First, policies or interventions can reduce the probability of a hazard occurring. A ship collision, for example, can be mitigated by establishing protective islands around piers. Road and rail traffic can be limited to reduce the chance of overloading. For example, in the United Kingdom, highway authorities must be notified when a vehicle weighing more than 44 tonnes is intended to be used on the public highway because many bridges are unsuitable for such loads.
Second, the probability that a bridge component will fail can be reduced, for example by strengthening piers and through regular inspections. Strengthening components is conceptually straightforward. However, it is often hard to judge what magnitude of load to design against, considering the unpredictability of extreme events over the lifetime of a bridge. Research on future climate trends would improve those assumptions. Besides inspections, sensors can also be installed on bridges to detect the early stages of damage, allowing prompt repairs before component failure.
Third, engineers can prevent the propagation of failures by improving connectivity between components so that loads carried by failed components can be redistributed over the rest of the structure12,13. Engineers need to better understand how loads would be redistributed for a wider range of bridge types.
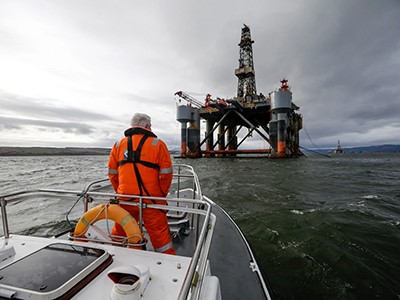
The world must rethink plans for ageing oil and gas platforms
Thankfully, the range of sensors and algorithms available for bridge monitoring has expanded greatly in recent years — as have markets for such systems, which are predicted to grow at an annual rate of 12% globally until 2033 (see go.nature.com/3wdjvmn). Ultrasound or ground-penetrating radar can be used to test material properties and to uncover defects and damages14. Fibre-optic sensors can monitor bridge vibrations and deformations15. And temperature and humidity sensors can acquire data on environmental and operational conditions.
Research on identifying early damage is mature16. But it’s harder to extrapolate from data to anticipate events that can trigger catastrophic failure. This requires computational simulations of possible failure scenarios — such as those being performed in the Pont3 project that we are currently working on. Such tools will help engineers to identify the most likely failure points and key parameters for monitoring, and to define optimal sensor locations to collect the maximum information with minimum resources.
Regulations will also be needed for bridge monitoring systems, which are currently deployed in an ad hoc way. And more must be done to develop automated and user-friendly processes for analysing monitoring data, which remain highly specialized. Researchers must collaborate with the bridge industry to make such practices more mainstream.
Learn from other sectors
Policymakers and the bridge industry should learn from sectors with stringent safety requirements, including the aviation and automobile industries. For instance, whereas a typical car is equipped with more than 100 sensors, and passenger aeroplanes have up to 10,000, most bridges are not monitored at all.
The installation and maintenance of monitoring systems are standardized and regulated in these other sectors; procedures and tools are optimized and tailored to the needs of the industry. Safety certification and inspection protocols for cars and planes are also well harmonized internationally, and the majority of professionals working in these industries are conscious of the importance of these measures. The bridge industry has a long way to go before attaining this level of regulation and clarity.
Overcoming these challenges collaboratively would help to reduce the risk of catastrophic collapse of bridges to a level that is as low as reasonably practicable. Safeguarding our bridges will help to build a resilient society in the face of an uncertain future.
Nature 629, 1001-1003 (2024)
doi: https://doi.org/10.1038/d41586-024-01522-6
References
-
Xu, F. Y., Zhang, M. J., Wang, L. & Zhang, J. R. J. Perform. Constr. Facil. 30, 04016030 (2016).